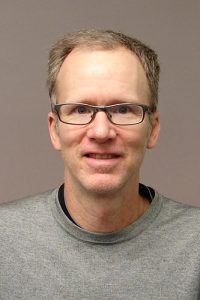
PhD
Professor
Chemistry
Biochemistry
Education
BS | Chemistry | University of Kansas | Lawrence, Kan. |
PhD | Chemistry | Northwestern University | Evanston, Ill. |
Research Area
Mechanisms of DNA damage by synthetic and naturally occurring antitumor agents, toxins and mutagens.
Research Description
The group employs the tools of synthetic organic chemistry, physical organic chemistry, biochemistry, biophysics, and molecular biology to study the molecular mechanisms of drug action. Students in the lab enjoy using a wide array of cutting-edge techniques to elucidate the products and mechanisms of the reactions that occur between biologically-active small molecules and their macromolecular targets in the cell.
Current research
DNA-damaging natural products as a source of new anticancer drugs
DNA serves as the molecular blueprint that directs all cellular operations. Accordingly, chemical modification of cellular DNA can have profound biological consequences. For example, many clinically-used anticancer drugs derive activity by causing DNA damage that kills rapidly dividing cancer cells. Accordingly, the development of new anticancer drugs will be advanced by the discovery of new fundamental mechanisms for the molecular recognition and chemical modification of DNA. Indeed such efforts are important to a variety of fields including medicinal chemistry, toxicology, and biotechnology.
Historically, structurally unusual natural products that possess potent biological activity have shown the potential to reveal mechanisms of DNA modification that are chemically unexpected and remarkably efficient. In addition, because of their potent bioactivity, natural products represent a rich source of pharmaceuticals. In fact, it has recently been estimated that more than 60% of the anti-infective and anticancer agents in current use or in advanced clinical trials are derived from natural products.
We are investigating the chemistry and biology of natural products that damage DNA by unusual chemical mechanisms. Below we show the structures of some natural products and their synthetic analogues that are currently under investigation in the lab. Some of these compounds generate radicals that lead to oxidative DNA damage, while others generate electrophilic species that alkylate DNA (for an overview of these DNA-damage pathways, please see the Reviews of Reactive Intermediate Chemistry chapter on the group’s Web site). In recent years, we have placed special emphasis on studies of leinamycin (Scheme below), a Streptomyces-derived natural product that gains exceptionally potent antitumor activity through its ability to simultaneously generate both DNA-damaging radicals and electrophiles by completely novel chemical pathways. In general, our studies with these natural products continue to reveal fantastically efficient chemical mechanisms for DNA damage that are beyond our wildest imaginings.
Hypoxia-selective antitumor agents
Solid tumors differ from most normal human tissue, in that they contain significant populations of oxygen-poor (hypoxic) cells. For this reason, medicinal chemists have long sought agents that selectively generate cell-killing reactive intermediates under hypoxic conditions. The compound 3-amino-1,2,4-benzotriazine 1,4-dioxide (tirapazamine) is the most promising hypoxia-selective antitumor agent discovered to date. The compound is currently undergoing a variety of phase I, II, and III clinical trials for the treatment of human cancers. The anticancer activity of this drug stems from its ability to selectively cause DNA damage in hypoxic tumor cells.
Upon entering cells, tirapazamine is enzymatically reduced to its radical form. In normally-oxygenated cells this radical undergoes relatively harmless back-oxidation to the starting drug. On the other hand, under hypoxic conditions (in tumor cells), the radical intermediate goes on to cause cell-killing DNA damage. The chemical mechanisms responsible for DNA-damage by tirapazamine are the subject of intense, ongoing studies in our group and others because understanding the mechanisms of clinically promising anticancer agents can lead to more effective therapeutic strategies and to the design of new, more potent analogues.
We are currently investigating the mechanisms of DNA damage by heterocyclic N-oxides and working to define the structure-activity relationships within this promising new class of drugs. This includes the study of naturally-occurring heterocyclic N-oxides such as myxin, iodinin, and carboxyquinoxaline di-N-oxide. Our work aims to test the hypothesis that bioreductively-activated N-oxides undergo homolytic fragmentation to release the well-known DNA-damaging agent hydroxyl radical. In addition, we are investigating the ability of tirapazamine and its metabolites to undergo secondary reactions with the initially-generated DNA radicals in a manner that mimics molecular oxygen to generate toxic DNA strand breaks and base labile lesions. Finally, we are employing heterocyclic N-oxides as a platform for the design of tumor-cell-selective alkylating agents, kinase inhibitors, and agents for fluorescent imaging of hypoxic cells in living organisms.
Discovery of small molecules that regulate the cellular activity of protein tyrosine phosphatases
Protein tyrosine phosphatases (PTPs) are cysteine-dependent enzymes that catalyze the hydrolytic removal of phosphate groups from tyrosine residues in proteins. PTPs, in concert with protein tyrosine kinases, play a central role in cell signaling by regulating the phosphorylation status and, in turn, the functional properties, of target proteins in various signal transduction pathways.
The cellular activity of some PTPs is regulated by endogenous hydrogen peroxide that is produced as a second messenger in response to extracellular stimuli such as insulin, epidermal growth factor, and platelet derived growth factor. Hydrogen peroxide inactivates PTPs via oxidation of the active site cysteine thiol residue to a sulfenic acid. In some cases, the cysteine sulfenic acid undergoes subsequent conversion to an active site sulfenyl amide or disulfide. Oxidative inactivation of PTPs inside cells is slowly reversed by reaction of the inactivated enzyme with biological thiols.
PTP inactivators are of widespread interest in medicinal chemistry and cell biology because of their potential to regulate or dysregulate important cellular signaling pathways. For example, PTP1B is a validated target for the treatment of type 2 diabetes. PTP1B is the major negative regulator of insulin signaling and inhibition of this enzyme prevents dephosphorylation of the insulin receptor and insulin receptor substrates, thus potentiating the action of insulin. We are currently investigating the fundamental chemical and enzymatic reactions underlying the redox regulation of PTPs. In addition, we are characterizing endogenous, dietary, and synthetic chemicals that modulate cellular PTP activity.
Selected Publications
Laciak AR, Korasick DA, Wyatt JW, Gates KS, Tanner JJ. (2019). Structural and biochemical consequences of pyridoxine-dependent epilepsy mutations that target the aldehyde binding site of aldehyde dehydrogenase ALDH7A1. FEBS J. 2019 Jul 14. doi: 10.1111/febs.14997. Epub ahead of print. [PubMed]
Nejad MI, Price NE, Haldar T, Lewis C, Wang Y, Gates KS. (2019). Interstrand DNA Cross-Links Derived from Reaction of a 2-Aminopurine Residue with an Abasic Site. ACS Chem Biol. 14(7):1481-1489. doi: 10.1021/acschembio.9b00208. [PubMed]
Nejad MI, Guo X, Housh K, Nel C, Yang Z, Price NE, Wang Y, Gates KS. (2019). Preparation and Purification of Oligodeoxynucleotide Duplexes Containing a Site-Specific, Reduced, Chemically Stable Covalent Interstrand Cross-Link Between a Guanine Residue and an Abasic Site. Methods Mol Biol. 1973:163-175. doi: 10.1007/978-1-4939-9216-4_10. [PubMed]
Gu LQ, Gates KS, Wang MX, Li G. (<a h). What is the potential of nanolock- and nanocross-nanopore technology in cancer diagnosis? Expert Rev Mol Diagn. 2018 Feb;18(2):113-117. org/10.1080/14737159.2018.1410060″>doi: 10.1080/14737159.2018.1410060. Review. [PubMed]
Korasick DA, Wyatt JW, Luo M, Laciak AR, Ruddraraju K, Gates KS, Henzl MT, Tanner JJ. (2017). Importance of the C-Terminus of Aldehyde Dehydrogenase 7A1 for Oligomerization and Catalytic Activity. Biochemistry. 56(44):5910-5919. doi: 10.1021/acs.biochem.7b00803. [PubMed]
Yang Z, Price NE, Johnson KM, Wang Y, Gates KS. (2017). Interstrand cross-links arising from strand breaks at true abasic sites in duplex DNA. Nucleic Acids Res. 45(11):6275-6283. doi: 10.1093/nar/gkx394. [PubMed]
Sarkar U, Hillebrand R, Johnson KM, Cummings AH, Phung NL, Rajapakse A, Zhou H, Willis JR, Barnes CL, Gates KS. (2017). Application of Suzuki-Miyaura and Buchwald-Hartwig Cross-coupling Reactions to the Preparation of Substituted 1,2,4-Benzotriazine 1-Oxides Related to the Antitumor Agent Tirapazamine. J Heterocycl Chem. 54(1):155-160. doi: 10.1002/jhet.2559. [PubMed]
Nejad MI, Shi R, Zhang X, Gu LQ, Gates KS. (2017). Sequence-Specific Covalent Capture Coupled with High-Contrast Nanopore Detection of a Disease-Derived Nucleic Acid Sequence. Chembiochem. 18(14):1383-1386. doi: 10.1002/cbic.201700204. [PubMed]
Punthasee P, Laciak AR, Cummings AH, Ruddraraju KV, Lewis SM, Hillebrand R, Singh H, Tanner JJ, Gates KS. (2017). Covalent Allosteric Inactivation of Protein Tyrosine Phosphatase 1B (PTP1B) by an Inhibitor-Electrophile Conjugate. Biochemistry. 56(14):2051-2060. doi: 10.1021/acs.biochem.7b00151. [PubMed]